Welcome to Energy Materials Research Group

Two-dimensional heterostructured catalysts for hydrogen generation
​
This research activity focuses on designing two-dimensional heterostructured materials such as MXene and transitional metal dichalcogenides (TMDs) for hydrogen evolution reaction (HER). Nanostructured materials with optimized morphology and surface chemistry will have many exposed active sites which can be beneficial for in-plane electron transfer during HER process. The performance of these catalysts are being tested at high current densities in a wide pH range from acidic to alkaline media.
​
MXene comprises transition metals carbides, carbonitrides, and nitrides, with the formula Mn+1XnT10 where M, X, T, and n represent transition metals, C/N, T represents surface functional groups (e.g. -OH, -F, -O) and n=1-3 respectively. MXene is synthesized by layered ternary carbide and nitride MAX phase. Different synthesis methods such as wet chemicals, hydrothermal etching, chemical vapor deposition, etc. are used for MXenes. MXene gaining interest due to its large surface area, metallic conductivity, and large-scale solution processability. The basal planes of MXenes have shown catalytic activity for HER where the HER activity can be further improved by controlling surface termination groups Tx and introducing new dopants.
​
Transition metal dichalcogenides (MX2, where M = transition metal, and X = chalcogen) have high catalytic activity, high chemical stability low cost, and ease of synthesis. However, TMD materials have limited affinity toward water in an aqueous electrolyte as it has coordinated sulfur edge sites rather than the basal plane.
Our group focuses on synthesis of 2D and heterostructured MXene and TMD-based heterostructures such as TMD/MXene via HF-free chemical etching and chemical vapor deposition methods. These materials are promising alternative for HER due to the rearrangement of the electronic structures of the active sites.
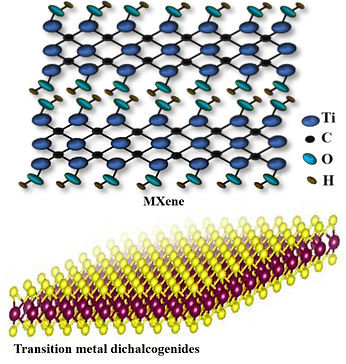
Porous materials-based membrane for CO2 capture and conversion
​
Reducing carbon dioxide (CO2) via electrochemical processes is a promising approach to address climate change and produce valuable chemicals and fuels by using suitable electrocatalysts. Covalent Organic Frameworks (COFs) have emerged as a promising platform for electrochemical carbon dioxide (CO2) reduction due to their well-defined structures and tunable properties. These crystalline materials, composed of organic building blocks linked by covalent bonds, provide a unique platform for efficient catalysis in electrochemical processes.
​
COFs offer a high degree of structural precision, allowing for the rational design of active sites for catalysis. The tunable nature of COFs allows for the creation of tailored pore structures, optimizing gas diffusion and reaction kinetics. CO2 molecules adsorb onto the active sites of the COF, facilitating interaction with the catalyst. The electrochemical reduction of CO2 takes place on the COF surface, leading to the formation of desired products such as methanol , CO, HCOOH, or hydrocarbons. Our group focuses on electrochemical CO2 reduction via various COFs such as metal porphyrin, metal free, Donor – acceptor COFs etc.

Electrocatalytic reactions on supported metal catalysts
​
This research activity focuses on study of surface reaction mechanisms such as oxygen reduction reaction, methanol oxidation, CO2 reduction on various supported metal nanoparticles catalysts. Reaction mechanisms at the solid/ liquid interface are studied using in situ infrared spectroscopy to get structural information of these surface absorbed metal nanoparticles. Understanding of these reaction mechanisms will help in development of better or alternative electrocatalysts.
Materials for pseudocapacitive applications
​
In last few decades, high energy density (~200 Wh/kg) property of lithium-ion batteries increases their demand in consumer electronic though they pose serious safety issues. In contrary, supercapacitors are safe to use with high power densities, rapid charging and long cycle life (>100,000) and hence widely used in heavy-duty vehicles, hybrid platforms and storing the regenerative braking energy of electric vehicles. The high capacitance of supercapacitor cells depends on the high surface area of the electrode materials and the voltage provided by the electrolyte used. The challenge to enhance the energy density of supercapacitor can be achieved by developing asymmetric hybrid capacitors where charge storage mechanism at one electrode is based on capacitive mechanism (electrode double layer capacitance; EDLC) and at the second electrode due to battery type fast faradaic reactions (pseudocapacitance). Our group focuses on development of materials (spinels, inverse spinels etc.) for aqueous and non-aqueous supercapacitor research applications using ionic liquids or organic liquids.